| |
[ Home ] [ Up ] [ Lecture Syllabus ] [ Notes: Set No. 1 ] [ Notes: Set No. 2 ] [ Notes: Set No. 3 ] [ Notes: Set No. 4 ] [ Lab Syllabus ] [ Study Guide for Lecture Course Final Exam ]
Plant Physiology
The following web page
represents a copy of my notes that formed the basis of lectures given during the
first portion of the Biology of Plants (BOT 1103) lecture course. Please refer
to your own notes, handouts, and to the textbook (Stern,
K., R., J. E. Bidlack, and S. H. Jansky. 2008. Introductory Plant Biology,
McGraw-Hill. 616 pp. - reading assignments are in the syllabus) for
additional information. This web page does not include information found in
various handouts and related materials (e.g., films, charts, overhead
projections, etc.) that you will receive during the course of the semester. You
will be evaluated over this information as well. If you note any errors in the
following document, I'd appreciate it if you would bring this to my attention.
Email address: mhuss@astate.edu.
- ENERGY AND METABOLISM
Metabolism - controlled capacity to acquire and use energy.
Energy - the capacity to do work.
Potential energy - stored energy.
Kinetic energy - energy of movement.
Heat - energy of random motion - not available for doing work.
Both potential and kinetic energy are available to do work, in other words, they are both
forms of free energy. Free energy is the energy in a system available for doing work.
Heat is a loss of free energy in the sense that it can not do work.
EXAMPLES:
- Water running out of a dam runs a turbine to make electricity or run a mill.
- In the covalent bonds of a glucose molecule.
- In an electron excited to a higher "orbit" or energy state by being struck with a photon of
sunlight.
First Law of Thermodynamics - Law of Conservation of Energy - total energy in the universe
is constant. Energy can be transformed from one form to another but not destroyed.
Second Law of Thermodynamics - In the universe as a whole the total amount of free energy is
declining (e.g., batteries run out of juice, a clock winds down). Free energy is being converted to
heat. Increasing randomness or disorder = ENTROPY.
Each time there is an energy transformation, some free energy is lost as heat.
CHEMICAL REACTIONS AND ENERGY
Endergonic processes or reactions (not spontaneous). For example:
CO2 + water + energy ==> glucose + oxygen.
Exergonic processes or reactions (spontaneous) For example:
glucose + oxygen ==> CO2 + water + energy
Activation energy needed to get many kinds of chemical reactions going (e.g., sparks in spark
plugs of an engine; heat and flames for firewood or charcoal briquettes).
Catalyst - lowers the activation energy without being consumed.
Enzymes - biological catalysts (protein). Protein has an active site - physical location on the surface of the enzyme where substrates bind to the protein.
E + S ======> ES =====> E + P
Lock and Key hypothesis - "older view of enzymatic action"
Induced-fit hypothesis - enzyme undergoes conformational changes in the course of bonding
with the substrate, which improves the fit and makes ES more reactive.
Cofactors - large nonprotein organic molecules that function as coenzymes (e.g., vitamins). Examples of nonprotein cofactors involved in electron and hydrogen ion transport are:
NAD+ + H+ + 2 e- ------> NADH nicotinamide adenine dinucleotide NADP+ + H+ + 2 e--------> NADPH nicotinamide adenine dinucleotide phosphate FAD+ + 2H+ + 2 e-- ------> FADH2 flavin adenine dinucleotide
Another group of molecules involved in electron transport are cytochromes. Cytochromes - contain metals (Fe, Cu) and generally function as carrier proteins bound to membranes.
CHEMICAL REACTIONS ARE CONTROLLED IN LIVING SYSTEMS
enzymes - mediate chemical reactions energy transfers are done in steps
Example: There are two ways to get from the top of a very tall building to the bottom floor. Keep
in mind that a person on the top floor of a building has a lot of potential energy relative to the
ground level.
- Jump out the window!
- Take the stairs and expend the potential energy a little at a time until
you get to the
bottom floor.
Both methods get you to the bottom floor but one method is destructive, while the other is not.
These two situations are analogous to uncontrolled vs. controlled energy transfers. Potential
energy transferred gradually so more work is done than heat.
In organisms, electron transport systems "intercept" excited electrons and make use of the energy
release. Series of oxidation (removal of electrons)/reduction (addition of electrons) reactions,
usually across a membrane.
ATP (adenosine triphosphate) delivers or picks up energy for almost all metabolic pathways.
ATP ===> ADP + Pi + 7.3 kilocalories of energy
PHOTOSYNTHESIS
Autotrophs - "self feeder". Examples include photosynthetic organisms (cyanobacteria, green
algae and plants).
Heterotrophs
- "other feeder". Examples include bacteria, some protists (e.g., amoebae,
paramecia, slime molds), fungi, non-photosynthetic parasitic plants (dodder, Indian pipe) and
animals.
Metabolism - ability to acquire and use energy.
Each time an energy transfer takes place, some energy is lost in the form of heat. Why are plants green? The presence of the pigment chlorophyll (a and b) in the thylakoid makes
the chloroplast appear green in color. Sunlight, within the visible spectrum of the electromagnetic
spectrum, is composed of different wavelengths or colors of light. When light hits a leaf, some of the light is absorbed, some passes through
the leaf (like light through a filter), and the rest is reflected away. The reason that leaves are green
is due to the fact that colors like red and blue are absorbed, while green and yellow are reflected
back to you. When light lands on the retina of your eye, your brain perceives this as the color
green. This phenomenon also explains why we see any object as being one color versus another.
Objects that are red reflect the red wavelengths of light and absorb all other wavelengths; objects
that are black reflect little light back, most light is absorbed; while those objects that appear to be
white reflect most of the light away, little light is absorbed.
Sunlight contains energy, which under the right conditions can be converted to other forms of
energy: e.g., chemical bond energy, electricity, kinetic energy, heat, etc.
Light possesses both wave-like and particle-like properties. Packets of energy called photons.
The shorter the wavelength of light, the more energy it contains per photon. The longer the
wavelength of light, the less energy it contains per photon. Wavelengths of light are measured in
very small units called nanometers.
Photosynthesis - a set of enzyme-mediated reactions in which light energy from the sun is converted into the chemical bond energy of found in glucose and ATP.
The overall chemical reaction for photosynthesis is:
6CO2 + 12H2
O + sunlight energy =====> C6 H12
O6 (glucose) + 6H2 O + 6O2
In eukaryotes, the organelle associated with photosynthesis is the chloroplast. Chlorophylls as and
b, the light absorbing pigments (along with accessory pigments like the
carotenoids) are
embedded in the thylakoid membrane that form the grana and membrane system (thylakoid
interior - pH of 5.0) within the chloroplast. The grana and thylakoid membrane system are
surrounded by the stroma (pH of 8.0).
Photosynthesis proceeds in two major steps: (1)
During the light dependent reactions [photchemical reactions], light energy is captured by pigments in chloroplasts and water is split to
yield hydrogen ions, electrons, and oxygen. When light strikes the electrons of the magnesium
atom, it gets excited. Electrons are jumped to a higher energy level and the energy transferred by
fluorescece (via photons of longer wavelengths and less energy) from one pigment molecule to
the next in an antennae complex until it is "captured" at the reaction center. This energetic electron is funneled to electron transport system
imbedded in the thylakoid membrane of the chloroplast. The electrons originate from the
breakdown of water (photolysis), which liberates hydrogen ions and oxygen. The electrons move
from one electron acceptor to another in the thylakoid membrane, starting at Photosystem II
(P680) and moving through Photosystem I (P700). The energetic electrons and hydrogen ions are used to generate ATP (by noncyclic
photophosphorylation and chemiosmosis - hydrogen passing through the channel protein, ATPase
or ATP synthase) and NADPH (from NADP+). The energy originally in light, is now present in
the chemical bonds associated with ATP and NADPH. The aforementioned method
(noncyclic photophosphorylation) occurs in eukaryotic plants - algae, mosses, ferns, conifers, and flowering plants.
(2)
The light dependent reactions [biochemical reactions] are associated with the thylakoid membrane system. The energy in ATP and NADPH is used during the light independent reactions, to "fix" the carbon in CO2 by producing a more complex carbohydrate. The series of
enzyme-mediated chemical reactions responsible for the capture and incorporation of "gaseous
carbon" into "solid" carbon compounds is called the CALVIN CYCLE (also referred to as C3
photosynthesis). The enzyme that initially starts off the process by reacting with
CO2
is called
ribulose bisphosphate carboxylase. This is the most abundant naturally-occurring protein
produced on the earth.
Know what PGA, GAL3P, and RuBP stand for!
In leafy plants, glucose is used to stored as sucrose or starch. Glucose is transported through
phloem (food-conducting vascular tissue). Another type of vascular tissue found in the leaves,
stems and roots is the xylem, which conducts water. Plants have a body design that enhances and
coordinates the process of photosynthesis. Above ground, these plants are highly branched to
increase the surface area for the absorption of sunlight and CO2. Below ground, plants have a
highly branched root system to anchor the plant and increase the surface area for the absorption
of water. Glucose and the stored chemical energy it contains are used to build more complex
organic compounds (e.g., polysaccharide, lipids, proteins, nucleotides). Energy, when needed
(e.g., germination of seeds and growth, at night when no photosynthesis is possible, in
underground roots), contained in glucose is liberated by mitochondria through aerobic respiration
. Other organisms take advantage of
the food produced by producers, such as plants to meet their own energy needs.
Photorespiration, C4, and CAM Photosynthesis
Leaves are organs of photosynthesis - responsible for harvesting light and carbon dioxide. Stomata open to allow for gas exchange (carbon dioxide in; water and oxygen out). Food is transported out of the leaf through the vascular system.
When weather is cool and water is readily available, the stomata open, causing concentrations of carbon dioxide to go up while oxygen levels go down.
High CO2 /low O2 ===> CO2 + RuBP (5 carbons) is converted by ribulose bisphosphate carboxylase to 2 molecules of PGA (3 carbons).
When days are hot and dry, stomata close causing concentrations of CO2 go down, while oxygen
goes up.
Low CO2
/high O2 ===> O2 + RuBP yields 1 PGA
(phosphoglycerate) and phosphoglycolic
acid.
In the mitochondria, phosphoglycolic acid is hydrolyzed to glycine (2 carbon amino acid). Two
glycine molecules combine forming CO2 (which may or may not be captured by RuBP carboxylase) and serine (3 carbon amino acid). Serine is converted to PGA at the expense of
ATP) which then enters the Calvin cycle. THIS PROCESS IS REFERRED TO AS PHOTORESPIRATION. Oxygen inhibits photosynthesis, because RuBP carboxylase can act as
an oxygenase - Warburg effect. Energy in the form of ATP and carbon are squandered.
C4 photosynthesis evolved to compensate for the negative effects of photorespiration.
Krantz Anatomy - veins encased by thick-walled photosynthetic bundle-sheath cells that are in
turn surrounded by tin-walled mesophyll cells (common in grasses, like cereal grains and sugarcane).
In the mesophyll cell, PEP carboxylase causes CO2 to combine with phosphoenolpyruvate (PEP)
to make oxaloacetic acid (OAA). This 4 carbon compound moves into the bundle sheath cell,
where carbon dioxide is dumped and moves into the Calvin cycle. C3 photosynthesis proceeds as
normal but in an environment saturated with carbon dioxide with minimal oxygen. Food is dumped
into the phloem, which is near the site of production, another advantage to these types of plants.
Advantages of C4 photosynthesis over C3 alone.
PEP carboxylase does not combine with oxygen, so this molecule does not interfere with
the harvesting of CO2. Rubisco is insulated from high concentrations of oxygen, because it is
only produced in the bundle sheath cells, and not in the mesophyll. C4 plants are more
efficient in their use of nitrogen, because they don't need as much Rubisco as C3 plants.
C4 photosynthesis is an adaptation against photorespiration that is common in hot dry
environments and it appears to have evolved independently in at least 17 angiosperm
families (including dicots and monocots). Common examples include, corn, sorghum,
sugarcane, millet, and pigweed. C4 photosynthesis is only more efficient than C3 plants in
conditions that are hot, bright, and dry.
Crassulacean Acid Metabolism (CAM)
Crassulacean Acid Metabolism (CAM) - unusual photosynthesis first discovered in a member of
the Crassulaceae (a group of succulent plants). Malic acid stored in vacuoles, because pH drops
to as low as 4 would otherwise damage the cell.
CO2 is harvested at night and stored in vacuoles as malic acid (4 carbon acid). During the day
CO2 is liberated into the chloroplast where it enters the Calvin cycle. CAM
photosynthesis is an
extreme adaptation to prevent water loss while maximizing photosynthesis. Common in desert and
tropical plants, including
Kalanchöe, pineapple, Spanish moss, two genera of ferns, cacti,
orchids, and Agave.
PLANT GROWTH REGULATORS (PLANT HORMONES)
-
Internal and external signals that regulate plant growth are mediated, at least in part, by plant
growth-regulating substances, or hormones (from the Greek word hormaein, meaning "to excite").
-
Plant hormones differ from animal hormones in that:
-
No evidence that the fundamental actions of plant and animal hormones are the same.
Unlike animal hormones, plant hormones are not made in tissues specialized for
hormone production. (e.g., sex hormones made in the gonads, human growth hormone -
pituitary gland)
-
Unlike animal hormones, plant hormones do not have definite target areas.
-
Animal hormones usually have specific effects, while those of plants seldom, if ever,
have specific effects (e.g., auxins stimulate adventitious root development in a cut shoot, or shoot
elongation or apical dominance, or differentiation of vascular tissue or etc.).
HORMONES ARE NECESSARY FOR, BUT DO NOT CONTROL, MANY ASPECTS
OF PLANT GROWTH AND DEVELOPMENT. - BETTER NAME IS GROWTH
REGULATOR.
-
THE EFFECT ON PLANT PHYSIOLOGY IS DEPENDENT ON THE AMOUNT OF
HORMONE PRESENT AND TISSUE SENSITIVITY TO HORMONE
-
THE EFFECT ON PLANT PHYSIOLOGY IS DEPENDENT ON THE AMOUNT OF
HORMONE PRESENT AND TISSUE SENSITIVITY TO HORMONE
FIVE MAJOR CLASSES OF PLANT HORMONES
- AUXIN (cell elongation)
- GIBBERELLIN (cell elongation + cell division - translated into growth)
- CYTOKININ (cell division + inhibits senescence)
- ABSCISIC ACID (abscission of leaves and fruits + dormancy induction of buds and
seeds)
- ETHYLENE GAS (promotes senescence, epinasty, and fruit ripening)
1. AUXIN => INDOLE-3-ACETIC ACID (IAA)
- First plant hormone discovered.
- During the 1870's, Charles and Francis Darwin studied coleoptiles (primary shoot) of canary grass and oats, both of which grow toward unidirectional
light (phototropism). In 1881, the Darwins published their findings in a book entitled
The Power of Movement of Plants. Conclusion: The growth of coleoptiles toward light is somehow
controlled by the tip of the coleoptile.
- Peter Boysen-Jensen (1913) - Cut tips of coleoptiles and placed an intervening piece of agar between the cut tip and the cut
coleoptile. The "influence" passed through the agar and reestablished the phototropic response.
He also concluded that "influence" was a water soluble substance, because "butter-barriers"
(water soluble substance could not pass through) and metal or mica barriers (not an electrical response)
did not allow the response to take place.
- Arpad Paál (1919) - Asymmetrical placement of cut tips on coleoptiles resulted in a bending of the coleoptile away
from the side onto which the tips were placed (response mimic the response seen in
phototropism).
- Frits Went (1926) - Demonstrated the diffusion of some chemical from the tips of coleoptiles into agar. Agar allowed
to take up "influence" from cut tips, then tips discarded, and blocks placed on cut coleoptiles,
response initiated.
- Phototropic response due to chemical produced by the tip, and not the physical presence of the
tip. Went named this chemical auxin (from the Greek word auxein, meaning "to grow").
Auxin influences phototropism: light striking one side of a coleoptile causes auxin the migrate to the
shaded side of the coleoptile, where it stimulates growth and causes growth toward light.
- The first auxin to be identified chemically was isolated from human urine. Functions of
auxins in animals is not known.
- IAA - naturally-occurring auxin 2,4-D (2,4-dichlorophenoxyacetic acid) and NAA
(naphthaleneacetic acid) - synthetic auxins.
- Synthetic auxins have been used as herbicides for killing dicot plants. Grasses are
monocots and symptoms are not as severe.
- Agent Orange - 1:1 ratio of 2,4-D and 2,4,5-T used to defoliate trees in Vietnam War.
Dioxin usually contaminates 2,4,5-T, which is linked to miscarriages, birth defects,
leukemia, and other types of cancer.
- Auxin manufactured by parenchyma cells of the cortex, pith, and vascular tissues.
Moves through the tissue polarly from the roots up into the stem (acropetal -
towards the shoot tip), and the tip of the shoot toward the base of the stem
(basipetal).
EFFECTS OF AUXIN
- Proper functioning of auxins depends on its interaction with calcium ion. What consequences
would calcium deficiencies have on a plant? Calcium stored in the vacuoles. Auxins produced by
the plant would be less effective, if calcium ions are lacking. Calcium activates
calmodulin, a
protein, which regulates many processes in plants, animals, and microbes.
- Acid-growth hypothesis - Cellular elongation in grass seedlings and herbs occurs because cell walls are loosened by the
activation of enzymes and drop in pH mediated by presence of auxin.
- Apical dominance - Axillary buds at the base of their petioles are inhibited near the shoot apex, but less so farther
from the tip. Apical dominance is disrupted in some plants by removing the shoot tip, causing the
plant to become bushy instead of cone-shaped (like a Christmas tree).
- Abscission - shedding of leaves during senescence (aging and death)
- Auxin interacts with other hormones (cytokinin, gibberellins) to prevent senescence. As auxin
levels drop, senescence begins, and production of ethylene and abscisic acid causes the formation
of an abscission zone (suberized cells, cellulase and pectinase). Deciduous plants lose their leaves every fall, while evergreens retain their
leaves for 2 or more years. Leaves (needles) on evergreens are not dropped all at once.
- Differentiation of vascular tissue - Vascular cambium is activated in the spring by auxin produced by young developing leaves.
- High auxin/low gibberellin promotes differentiation of xylem.
- Low auxin/low gibberellin promotes differentiation of phloem.
- Nonhormonal factors + auxin - Auxin + small amounts of sucrose (2%) favors differentiation of xylem.
Auxin + moderate amounts of sucrose (3%) favors differentiation of xylem and phloem.
Auxin + large amounts of sucrose (4%) favors differentiation of phloem.
- Fruit development - Seeds produce auxins which stimulates fruit development and ripening. Parthenocarpic (seedless)
fruits can be produced in tomato and cucumbers. The achenes of strawberry produce
auxins, without these the receptacle of this aggregate fruit will not expand. That's why there are "no
seedless" strawberries.
- Adventitious roots - Cuttings can be stimulated to produce adventitious roots by dipping them in auxin (powder or
mixed as a lanolin paste).
2. GIBBERELLINS
- In 1930's, Ewiti Kurosawa and colleagues were studying plants suffering from
bakanae, or
"foolish seedling" disease in rice. Disease caused by fungus called, Gibberella
fujikuroi, which was stimulating cell elongation and division. Compound secreted by fungus could cause bakanae
disease in uninfected plants. Kurosawa named this compound gibberellin.
- Gibberella fujikuroi also causes stalk rot in corn, sorghum and other plants. Secondary
metabolites produced by the fungus include mycotoxins, like fumonisin, which when ingested by
horses can cause equine leukoencephalomalacia - necrotic brain or crazy horse or hole in the
head disease. Considered to be a carcinogen.
- Many different types of gibberellins - many are probably inactive precursors of active forms of
gibberellins (or gibberellic acid).
EFFECTS OF GIBBERELLINS
- Stimulation of shoot elongation by GA vs. auxin
- GA controls internode elongation in mature regions of trees, shrubs, and a few grasses,
whereas IAA regulates elongation in grass seedlings and herbs.
- GA induces cellular division and cellular elongation; auxin induces
cellular elongation alone.
- GA-stimulated elongation does not involve the cell wall acidification characteristic of
IAA-induced elongation
TAKE HOME MESSAGE - Different hormones can elicit similar effects via different
mechanisms.
- Flowering - Phenomenon of bolting (rapid elongation of stem during flowering process of rosette [short
internodes, leaves pack together like a cabbage]. Bolting is common in biennials like radish,
cabbage, and carrot.
- Seed Germination - Especially in cereal grasses, like barley. Not necessarily as critical in dicot seeds.
- Gibberellins can break dormancy or substitute for a cold treatment in some plants (e.g., tobacco,
lettuce, oats).
- Fruit Formation - "Thompson Seedless" grapes grown in California are treated with GA to increase size and
decrease packing.
3. CYTOKININS
- Gottlieb Haberlandt in 1913 reported an unknown compound that stimulated cellular division.
- In the 1940s, Johannes van Overbeek, noted that plant embryos grew faster when they
were supplied with coconut milk (liquid endosperm), which is rich in nucleic acids.
- In the 1950s, Folke Skoog and Carlos Miller studying the influence of auxin on the growth of
tobacco in tissue culture. When auxin was added to artificial medium, the cells enlarged but did
not divide. Miller took herring-sperm DNA. Miller knew of Overbeek's work, and decided to
add this to the culture medium, the tobacco cells started dividing. He repeated this experiment
with fresh herring-sperm DNA, but the results were not repeated. Only old DNA seemed to
work. Miller later discovered that adding the purine base of DNA (adenine) would cause the cells
to divide.
- Adenine or adenine-like compounds induce cell division in plant tissue culture. Miller, Skoog and
their coworkers isolated the growth facto responsible for cellular division from a DNA preparation
calling it kinetin which belongs to a class of compounds called cytokinins.
- In 1964, the first naturally occurring cytokinin was isolated from corn called
zeatin. Zeatin and
zeatin riboside are found in coconut milk. All cytokinins (artificial or natural) are chemically similar
to adenine.
- Cytokinins move nonpolarly in xylem, phloem, and parenchyma cells. Cytokinins are found in
angiosperms, gymnosperms, mosses, and ferns. In angiosperms, cytokinins are produced in the
roots, seeds, fruits, and young leaves.
- For historical overview of cytokinin, see the following article:
1955 Kinetin Arrives.pdf.
Article courtesy of Richard Amasino. Dr.
Amasino is a Distinguished Professor of Biochemistry at the University of
Wisconsin-Madison.
EFFECTS OF CYTOKININS
- Cellular Division - Cytokinins hasten the transition of cells from the G2 phase to mitosis. This effect requires the
presence of auxins.
- Effects on cotyledons - Cytokinins promote cellular division and expansion in cotyledons. Increases cell wall plasticity but
does not involve wall acidification (as the case with auxins).
- Organogenesis
- Cytokinins and auxin affect organogenesis.
- High cytokinin/auxin ratios favor the formation of shoots.
- Low cytokinin/auxin ratios favor the formation of roots.
- Senescence
- Cytokinins delay the breakdown of chlorophyll in detached leaves by preventing the genes that
produce chlorophyll from being turned off.
- The presence of calcium ions Ca2+ increases the sensitivity of tissues to cytokinins.
4. ETHYLENE
- In the 1800s, it was recognized that street lights that burned gas, could cause neighboring plants to
develop short, thick stems and cause the leaves to fall off. In 1901, Dimitry Neljubow
identified that a byproduct of gas combustion was ethylene gas and that this gas could affect plant
growth.
- In R. Gane showed that this same gas was naturally produced by plants and that it caused faster
ripening of many fruits.
- Synthesis of ethylene is inhibited by carbon dioxide and requires oxygen.
- Fruit ripening
- Only climacteric fruits (tomato, apple, banana, orange) respond to ethylene, which is
characterized by increased rates of respiration, softening of fruit tissue, changes in sweetness and
color.
- Flowering
- Ethylene inhibits flowering in most species, but promotes it in a few plants such as pineapple,
bromeliads, and mango. Spraying plants with ethephon, a compound that breaks down into
ethylene, using fires or presence of ripening fruits can stimulate this process.
- Abscission The increased production of ethylene at the abscission zone triggers the breakdown of the middle
lamella, and thereby initiates abscission in leaves and fruits.
- Sex Expression
- Ethylene and gibberellins can determine the sex of a flower. Cucumber buds treated with ethylene
become carpellate (female) flowers, whereas those treated with gibberellins become staminate
(male) flowers.
- Stem Elongation
Shaking a plant increases ethylene production which in turn causes
thigmomorphogenesis. The
plants form short thick stems. Effect is mediated by ethylene.
- Roots and shoots
- Ethylene buildup in the roots, stimulates production of pectinases and cellulase which helps create
many intercellular spaces characteristic of hydrophytes. Even though ethylene production is reduce
in waterlogged (anaerobic) soil, what little that is produced is trapped and elicits the above
response. In shoots, ethylene causes parenchyma cells on the upper side of the petiole to expand
and point the leaf down (epinasty).
5. ABSCISIC ACID (ABA)
- In 1940s, scientists started searching for hormones that would inhibit growth and development,
what Hemberg called dormins. In the early 1960s, Philip Wareing confirmed that dormin to a bud
would induce dormancy. F.T. Addicott discovered that this substance stimulated abscission of
cotton fruit. he named this substance abscisin. (Subsequent research showed that ethylene and
not abscisin controls abscission). Abscisin is made from carotenoids and moves nonpolarly through plant tissue.
- Closure of Stomata
- During drought, leaves make large amounts of ABA which causes stomata to close. Potassium
ions leaving guard cells cause water move by osmosis as well. Cells lose turgor pressure causing
the stomata to close.
- Bud and Seed Dormancy
ABA induces bud and seed dormancy in many plant species and may work in conjunction with
other plant hormones (IAA and cytokinins).
ABA counteracts the stimulatory effects of other hormones. These effects may occur because
ABA is a calcium antagonist.
HOW PLANTS RESPOND TO ENVIRONMENTAL STIMULI
I. Tropisms - plant growth toward or away from a stimulus such as light or gravity.
PHOTOTROPISM
Blue light less than 500 nm in wavelength induces phototropism. IAA accumulates on the shaded
side of the shoot and causes elongation of cells. Consequently the shoot bends toward the light.
GRAVITROPISM
Charles and Frances Darwin discovered that plants require the root cap in order to respond to
gravity. Sedimentation of starch-laden amyloplasts in root cap cells without amyloplasts are still
able to respond to gravity. Role of amyloplasts uncertain in perception of gravity. Role of
amyloplast uncertain in perception of gravity. Ca++ and auxin are involved in bending of roots
toward gravity. Ca++ and IAA inhibits cell elongation in roots. So up the side of the root where
IAA does not accumulate, elongates and causes the root to bend towards gravity. Roots are
positively gravitropic and grow downward, whereas stems are negatively gravitropic
and grow
away from the pull of gravity.
HYDROTROPISM - mediated by the root cap, movement of roots toward water in soil.
THIGMOTROPISM - growth response to touch, tendrils (garden peas and beans) and stems
(bindweed and morning glory) wind around on object they are using for support. IAA and ethylene are involved.
II. Nastic Movements - response to environmental stimuli that are independent of the
direction of the stimulus. Pre-determined response.
SEISMONASTY - a nastic movement resulting from contact or mechanical shaking.
- Sensitive plant - Mimosa pudica, touching the leaf causes the leaflet to fold and the petiole to
droop. Touching the leaf initiates an electrical signal that moves along the petiole.
Cells become more permeable to K+ and other ions. Parenchyma cells (motor cells) are located in a joint-like structure
(pulvinus) located at the base of each leaflet and the petiole.
Movement of ions out of motor cells decreases the water potential in the surrounding extracellular space via osmosis.
Loss of water causes the cells to shrink creating movement.
- VENUS'S FLYTRAP.
Three hairs located on the surface of each of the trap leaves. An insect triggering the hairs causes
an electrical signal to travel into the modified leaf tissue. Hydrogen ions are transported to cell
walls of the outer epidermal cells along the outside of the hinge. These acidified cells enlarge. The
epidermal cell walls along the inner side of the hinge are not acidified and do not enlarge. The
resulting tension causes the trap to close.
- Alternate hypothesis suggests that mesophyll cells of trap are compressed, causing the trap to be
under tension. The tension is released when the insect touches the hair triggers.
NYCTINASTY - sleep movements: prayer plant - lower leaves during the day and raises leaves
at night; Oxalis (shamrock) and legumes.
SEASONAL RESPONSES OF PLANTS TO THE ENVIRONMENT
Photoperiodism - (ability of plants to measure seasonal changes in daylength).
Flowering in many plants is induced by length of day and night.
Day-neutral plants flower with no regard to photoperiod.
Short-day plants flower only if light periods are shorter than some critical length.
Ragweed plants flower when exposed to 14 hours or less of light per day. Asters, poinsettias, potatoes, and
goldenrods (FALL PLANTS). [LONG-NIGHT PLANTS]
Long-day plants flower in the spring or early summer; they flower only if light periods are longer
than a critical length, which is usually 9-16 hours. Lettuce, radish, gets, clover, corn, and
iris [SHORT-NIGHT PLANTS]
Interrupt the dark period of a long night, for as little as 1 minute and a long night plant will not
flower, but a short night plant will flower. Phytochrome appears to be the light receiving pigment
in the leaves that controls photoperiod. Phytochrome exists in two interconvertible forms, Pr
(inactive form) and Pfr (active form).
Sunlight contains more red light than far-red light.
Pr ===> red light ===> Pfr (promotes flowering in short night plants, but inhibits flowering in long
night plants)
Pfr ===> far-red light (more slowly converted in dark) ===> Pr
Phytochrome responses + internal clock regulate photoperiod and flowering.
Florigen - hypothetical hormone that is produced by leaves and induces a flowering response.
Evidence exists that it exists, but no one has ever isolated it.
Phytochrome also is important in seed germination in some plants. Lettuce and weed seeds.
Presence of Pr inhibits germination, while its conversion to Pfr in red light induces germination.
Red light ===> germination
Far-red light ===> no germination
Red ===> far-red ===> red ===> germination
Red ===> far-red ===> red ===> far-red ===> no germination
Those seeds not buried deep in the ground get exposed to red light, and this signals germination.
Etiolated seedlings convert to normal stem growth when grown in the light and not the dark.
Response is mediated by phytochrome. Pr converts to Pfr and normal growth is restored.
Final note: Photoperiod also controls dormancy and leaf senescence in many
plants.
CIRCADIAN RHYTHMS
Regular daily rhythms are called circadian rhythms. Opening of flowers, folding of leaves, stomatal
opening, protein synthesis, secretion of nectar, hormone synthesis cellular division etc...
Biological clock of a plant is probably set by phytochrome, and rhythmic changes in transport and
metabolism in cells due to daily activities.
Genetic Engineering
and Bioengineering
- Transformation - introduction of a new gene into a bacterium, fungus,
plant or animal by some mechanism. (i.e., bombardment, electroporation,
virus- or bacteria-mediated gene transfer).
Transgenic organisms are produce by:
- Addition of new genes (to express a new gene product).
- Suppression of a gene or a gene product.
Potential benefits of transgenic organisms (GMOs
- Genetically-modified organisms)
Genetic engineering can produce organisms
that are:
- able to synthesize oils, starches, hormones (e.g., bacteria that produce
human insulin for use by diabetics; plants can synthesize special proteins) and
bioplastics
- edible vaccines from vegetables and milk
- able to synthesize enzymes for food processing and other uses
- more nutritious foods (e.g., plants with a higher protein content, and
wider profile of essential amino acids - methionine-rich beans or
lysine-rich corn; golden rice to help enrich the diets of those not able to
eat foods rich in beta-carotene or Vitamin A to prevent blindness caused by
a nutritional deficiency)
- plants able to fix their own nitrogen for growth
- freeze resistant plants
- pest resistant plants
- herbicide resistant plants
- disease resistance in animals and plants
- gene therapy to help cure certain diseases linked to the under or over
abundance of a protein product by a genetic disorder.
Potential problems
- Allergies to transformed plant and animal products.
- Accidental movement of novel genes into wild relatives from domesticated
plants and animals.
- Consumer resistance to using genetically-modified products, especially
food and drugs.
- Ethical and moral considerations. (e.g., exploitation of genetic
resources for personal gain).
EXAMPLES OF GENETIC ENGINEERING
Bioluminescent Tobacco (Are Christmas Trees Next? -
http://www.biocrawler.com/encyclopedia/Bioluminescence)
From
http://www.biocrawler.com/encyclopedia/Image:Glowing_tobacco_plant.jpg:
"An image of a tobacco plant
(SEE BELOW) which has
been genetically engineered to express a gene taken from
fireflys (specifically: Photinus pyralis) which produces
luciferase. The image is an "autoluminograph" produced by
placing the plant directly on a piece of Kodak Ektachrome 200
film. When the plant is watered with a luciferin containing
nutrient medium, tissue specific luminescence is observed. This
image was first published in a November 1986 issue of the
journal Science in a paper titled "Transient and stable
expression of the firefly luciferase gene in plant cells and
transgenic plants".
[1] (http://www.pgec.usda.gov/Ow/A10-1986OwetalScience.pdf)
by David W. Ow, Keith V. Wood, Marlene DeLuca, Jeffrey R. de
Wet, Donald R. Helinski and Stephen H. Howell. The research was
funded by grants from the US Dept. of Agriculture and the
National Science Foundation."
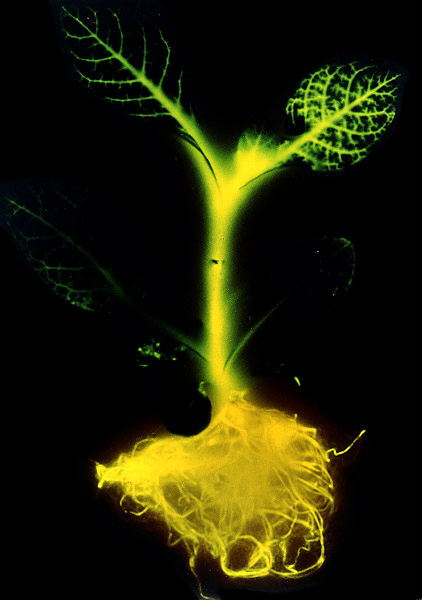
For more information on Genetically-Modified Plants visit:
This web page was assembled by Dr. Martin J. Huss - Last modified on
November 6, 2007
| |
|